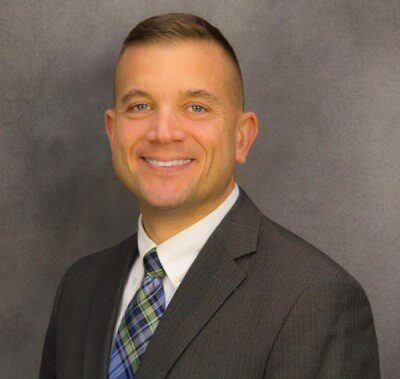
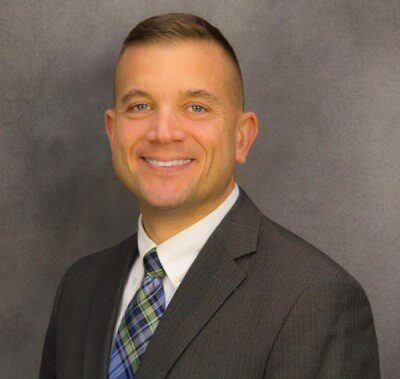
Paul Glotfelter’s first days in Magnus Egerstedt’s lab didn’t go as planned.
One afternoon a colleague gave Glotfelter a tiny robot that wasn’t working correctly and asked him to perform some tests. He scooped up the machine, walked out of the lab, and sat down at his desk in the next room.
Suddenly, it roared back to life with no notice, twitching around on its little rubber wheels. Glotfelter hadn’t given it a command or tinkered with its code.
“It seemed to have a mind of its own,” he remembers with a laugh.
“I was new, so I was a bit disturbed and annoyed. I put it on its side to keep it from moving. That’s when things really got confusing.”
Unbeknownst to Glotfelter, his colleagues in the neighboring lab were running an experiment with a swarm of nearly a dozen robots. The server, however, kept referencing an additional machine, trying to move it into the formation.
It took a few minutes before the mystery was solved. “We had a zombie robot on Paul’s desk,” says Egerstedt.
Two years later, Glotfelter is very comfortable with someone else controlling his robots. The rest of the world is now invited.
This month Georgia Tech opens the Robotarium, a $2.5 million lab funded by the National Science Foundation (NSF) and Office of Naval Research.
The 725-square-foot facility houses nearly 100 rolling and flying swarm robots that are accessible to anyone.
Researchers from around the globe can write their own computer programs, upload them, then get the results as the Georgia Tech machines carry out the commands. Egerstedt’s team also sends video evidence of the experiment.
Egerstedt says the concept is easy: robots for everyone.
“Building and maintaining a world-class, multi-robot lab is too expensive for a large number of current and budding roboticists. It creates a steep barrier for entry into our field,” says Egerstedt, the Julian T. Hightower Chair in Systems and Controls and a professor in the School of Electrical and Computer Engineering.
“We need to provide more access in order to continue creating the next generation of robots and robot-assisted technologies. The Robotarium will allow that at an unprecedented scale.”
No other university has such a facility.
It’s located in the Van Leer Building in the heart of Georgia Tech’s campus. Motion capture cameras cling from the ceiling and peer down at the lab’s centerpiece: a white, bowl-shaped arena that looks like a 12’ x 14’ hockey rink. That’s where up to 80 palm-sized, rolling robots scoot around the surface.
They automatically activate when given a program from someone in the room or a remote coder in a different state or country. Once it finishes the experiment, the swarm autonomously returns to wireless charging slots on the edge of the rink and waits to be activated for its next mission.
The lab is currently set up for the 3-D-printed rolling machines. In a few weeks, autonomous quadcopters the size of small dinner plates will whiz through the air for remote flying experiments (a retractable net will keep them from slamming into walls or people if things unexpectedly get out of control).
A large window allows curious onlookers to watch the organized chaos.
“The Robotarium is a terrarium for robots,” says Egerstedt.
“We wanted to create a space where anyone, at any time of the day or night, can walk past the lab and see robots in action. Too many robot labs are hidden away behind closed doors.”
That’s exactly how Egerstedt’s team worked for the last year and a half. They experimented in an unassuming space across campus in Technology Square, using a tabletop version of the Robotarium.
The mini surface allowed them to iron out kinks and identify potential problems with open-access robotics. For instance, what if someone purposely uploaded code that would cause the bots to collide and demolish each other?
“That’s why we created algorithms that wrap a virtual barrier around each machine to prevent collisions,” says Siddharth Mayya, a Ph.D. student in the lab. “We also had to worry about hackers.”
A portion of the NSF grant allowed the team to develop processes that protect the system from cyber threats.
Not everything always went smoothly. Ph.D. student Li Wang once hit a button that sent his swarm of quadcopters shooting toward the ceiling.
“It rained robots that day,” Wang recalls.
Another time, a rolling swarm descended on the same charging station at the same time. The robots literally fought for a spot until they reached the metal rail, which shorted them out and sprayed sparks across the room.
That’s why the Robotarium’s charging stations are now wireless.
So far more than 100 research groups have logged on and used the mini-version.
Most are roboticists without access to swarm technology. Others are biologists. One team chose to use robots, instead of computer simulations, to better understand how ants interact with each other when choosing a new queen.
Egerstedt thinks the new facility will foster more collaboration within the robotics community, allowing scientists and engineers to share their findings more widely and build on successes.
The open access setting will counter the lack of resources that sometime stands in the way of research.
“I want to do for robotics what MOOCs (massive open online courses) have done for education — now anyone who knows how to code can work with robots,” says Egerstedt, who is also the executive director of Georgia Tech’s Institute for Robotics and Intelligent Machines.
He already has a new recruit. This past April, a group of fifth graders stopped in for a tour. Egerstedt saw one of the 10-year-olds stuffing one of the Georgia Tech robots into his pocket while turning to leave.
“I asked him why he took it,” Egerstedt remembers. “He said he wanted to make it better.”
How?
“By adding a flamethrower.”
- by Jason Maderer, Georgia Institute of Technology
Woodruff School graduate students Allison Mahvi, advised by Dr. Srinivas Garimella, and Megan Tomko, advised by Dr. Julie Linsey, have been selected as American Society of Mechanical Engineers (ASME) Graduate Teaching Fellows for the 2017-2018 academic year.
The ASME Graduate Teaching Fellowship Program is a collaboration between ASME and mechanical engineering departments to encourage outstanding doctoral candidates in mechanical engineering education (and related engineering fields), particularly women and minorities. Awards are made for a maximum of two years and fellows are selected/renewed annually by the ASME Board of Education.
Past Woodruff School Graduate Teaching Fellows include:
1992 - Margueritte Patricia Brackin, Ph.D ‘97
1994 - Johné M. Parker, Ph.D, ‘96
1995 - Christopher C. Pascual, Ph.D ‘99
1996 - Stacey Angela Dixon, Ph.D ‘00
1998 - Laura Atkinson Schaefer, Ph.D ‘00
1999 - Stephanie Kladakis, Ph.D ‘02
2000 - Wayne Johnson, Ph.D ‘04
2002 - Phillip A. Vogelwede, Ph.D ‘04
2003 - Susan Stewart, Ph.D ‘03
2004 - Nathan D. Masters, Ph.D ‘06
2005 - Anne-Marie Lerner, Ph.D ‘08
2006 - Joshua Vaughn, Ph.D ‘08
2011 - Ashley Bernal, Ph.D ‘11
2012 - Brian Fronk, Ph.D ‘14
2013 - Thomas Stone, Ph.D ‘12
ASME is a not-for-profit membership organization that enables collaboration, knowledge sharing, career enrichment, and skills development across all engineering disciplines, toward a goal of helping the global engineering community develop solutions to benefit lives and livelihoods.
The Georgia Research Alliance has announced the inaugural award of the “Dr. J David Allen Family Seed Grant Program in Cellular Manufacturing.” The project is entitled "Microfluidic Technologies for Improved Engineering of iPSCs and their Applications to Treating Corneal and Lymphatic Diseases." The team consists of Dr. Todd Sulchek (Georgia Tech), Young-sup Yoon (Emory), and James Lauderdale (UGA).
The goal of the project is to analyze and validate a mechanical method to deliver "reprogramming factors" to human somatic cells to create induced pluripotent stem cells (iPSCs). The microfluidic/mechanoporation method will achieve higher delivery efficiency of these necessary factors, maintain cell viability, and will be designed to be high throughput. The resulting iPSCs will be validated through in vivo studies and tested for their ability to differentiate into lymphatic endothelial cells (for treatment of lymphedema and cancer) or corneal epithelial cells (for treatment of eye disorders).
The team will receive $90,000 in funding from the GRA made possible through a generous gift by Dr. J. David Allen to support research in cellular manufacturing across Emory University, the University of Georgia and Georgia Tech. The intent of Dr. Allen’s gift is to accelerate collaboration, stimulate innovation, and enhance the reputation of these three GRA partner universities.
Automation and Mechatronics, one of twelve Research Area Groups in the Woodruff School of Mechanical Engineering, has made the decision to change it's name to further support the group's vision, mission and strategy.
"The Automation and Mechatronics (A&M) Research Area Group is proud to announce the change of its RAG name to Automation, Robotics and Control (ARC). The RAG has a long history of conducting world-class research in system dynamics, machine design, and controls for advanced mechatronics and automation systems," says Dr. Jun Ueda, ARC's Research Area Group Chair. "Recently Robotics has become the RAG's focal area of research, particularly with the addition of the new faculty with robotics expertise. ARC faculty members are working closely with the Institute for Robotics and Intelligent Machines (IRIM). We hope that the new RAG name represents our faculty's contribution to this exciting field more precisely."
Research in the Automation, Robotics and Control area varies from fundamental research in control theory to the conception, design, and prototype evaluation of innovative mechatronics systems and applications to automation. Research in mechatronics focuses on the fusion of mechanical and electrical disciplines in modern engineering processes, aimed at achieving a cost-effective, optimal balance between mechanical structure and their overall control. Research topics include active and passive damping, adaptive learning and robust control of systems with uncertainty, automated manufacturing and demanufacturing, fuzzy and neural networks for control and identification, precision engineering and motion control, multimedia technology, intelligent sensors and acutators, vision-based motion control, and teleoperation.
Each year, Institute Diversity publishes Faces of Inclusive Excellence. The publication recognizes a diverse group of faculty, staff, and students who are committed to advancing a culture of inclusive excellence at Georgia Tech and who have distinguished themselves in their research, teaching, and/or service. This year's honorees include Woodruff School Associate Professor Baratunde Cola and graduate students Caleb Amy, Arkadeep Kumar, and Akanksha Menon.
The National Science Foundation (NSF) has awarded nearly $20 million to a consortium of universities to support a new engineering research center (ERC) that will work closely with industry and clinical partners to develop transformative tools and technologies for the consistent, scalable and low-cost production of high-quality living therapeutic cells. Such cells could be used in a broad range of life-saving medical therapies now emerging from research laboratories. Woodruff School faculty members Drs. Andrei Fedorov, Andrés Garcia, and Robert Guldberg are among the PIs leading the NSF Engineering Research Center for Cell Manufacturing Technologies.
Led by the Georgia Institute of Technology, the NSF Engineering Research Center for Cell Manufacturing Technologies (CMaT) could help revolutionize the treatment of cancer, heart disease, autoimmune diseases and other disorders by enabling broad use of potentially curative therapies that utilize living cells – such as immune cells and stem cells – as “drugs.” Examples of these highly promising therapies include T cell-based immunotherapies for blood cancers, such as the one developed at the University of Pennsylvania and approved in August by the U.S. Food & Drug Administration, and a gene-modified stem cell therapy recently approved in Europe for a form of the so-called “bubble boy” syndrome.
To facilitate the widespread application of these cutting-edge emerging treatments, CMaT will develop robust and scalable technologies, innovative analytical tools, and engineering systems that will enable industry and clinical facilities to reproducibly manufacture efficient, safe and affordable cell-therapy products. The center, one of four ERCs announced September 12 by the NSF, will also develop improved models for a robust supply chain, storage and distribution system for these therapeutic cell products.
“For over 30 years, NSF Engineering Research Centers have promoted innovation, helped to maintain our competitive edge, and added billions of dollars to the U.S. economy,” said NSF Director France Córdova. “They bring together talented innovators and entrepreneurs with resources from academia, industry and government to produce engineers and engineering systems that solve real-world problems. I am confident that these new ERCs will strengthen U.S. competitiveness for the next generation and continue our legacy of improving the quality of life for all Americans.”
In addition to the consistent manufacture of cell-based therapies, the public-private CMaT initiative will also help develop a skilled, diverse and inclusive bio-manufacturing workforce through extensive education and training activities at the K-12, technical college, undergraduate, graduate and postdoctoral levels.
Living cells become “drugs”
“Unlike pharmaceuticals and other products now used in medical treatments, cells are living entities whose properties can significantly change depending on nuances in the way they are grown, stored or otherwise manipulated,” said Krishnendu Roy, director of CMaT and the Robert A. Milton chair professor in the Wallace H. Coulter Department of Biomedical Engineering at Georgia Tech and Emory University. “The center will develop new engineering tools and scalable methods to better characterize, expand, differentiate, separate, transport and store high-quality cells so they provide consistent therapeutic effects, allowing them to be used in standardized therapies by clinicians to serve large numbers of patients worldwide.”
Beyond Georgia Tech, the center will include major partners – the University of Georgia, the University of Wisconsin-Madison and the University of Puerto Rico, Mayaguez Campus – as well as affiliate partners such as the University of Pennsylvania, Emory University, the Gladstone Institutes and Michigan Technological University. Additional international academic partners, as well as industry and the U.S. national laboratories, will also be critical collaborators in the effort.
Moving discoveries into application
“Georgia Tech has a long history of building collaborative partnerships with industry, the national labs and other research universities. With the support of the NSF and this new ERC, we will be able to capitalize on expertise in multiple areas, taking transformative research from the laboratory to practice much more quickly,” said Georgia Tech President G. P. “Bud” Peterson. “The Center for Cell Manufacturing Technologies will also help us educate, train and prepare the workforce in a new industry, thereby continuing to strengthen the U.S. economy.”
Clinical trials have already established the effectiveness of several cell-based therapies and many other trials are underway. But for these exciting therapies to advance into broad healthcare use, the cells will have to be produced in much larger quantities and with more consistent quality than is now available. There are also very few, if any, established industry standards for analytics and processes in cell manufacturing, which hinders consistent production of safe and efficacious cells. Another key limitation identified by industry is the need for a highly-trained workforce.
CMaT would address these barriers through transformative innovations that build upon a series of earlier efforts, including the National Cell Manufacturing Consortium (NCMC) roadmap, infrastructure established at Georgia Tech with support from the Marcus Foundation, quality and other standards programs from the National Institute of Standards and Technology (NIST) and independent industry-led bodies, and translational activities by industry, entrepreneurs and other partners.
The NSF’s multidisciplinary engineering research centers address unique, complex engineering challenges by stimulating knowledge and tech transfer between different sectors, from electronics to energy to infrastructure. Each center takes on a specific engineering research challenge.
“The overall goal of the NSF Engineering Research Centers program is nothing less than to revolutionize engineering research and education in the United States,” said Dawn Tilbury, NSF assistant director for engineering. “We look forward to the exciting advances and outcomes in these important areas.”
Accelerating clinical trials
Beyond established cell-based therapies, the work of CMaT should accelerate the development of new therapies and the testing needed to bring them into the clinic, said Steven Stice, director of the University of Georgia’s Regenerative Bioscience Center (RBC). Regenerative medicine applications could offer new ways of treating diseases for which there are now essentially no treatments, including Parkinson's, Alzheimer’s, heart disease and stroke.
“There are a significant number of cell therapy clinical trials and investments in the field,” Stice said. “But there is little or no investment in a set of consistent standardization methods to optimize how these therapies should work. For instance, we know that cell therapies will improve human health, but right now it’s difficult to guarantee that each dose produced will be as potent as the next. The work done by CMaT researchers will help solve some of these problems.”
The University of Pennsylvania develops cellular therapies and has conducted more than 40 clinical trials of cell-based therapies, including those for engineered T cell therapies and chimeric antigen receptor (CAR) T cells. An example is recently-approved treatment for relapsed and refractory acute lymphoblastic leukemia in pediatric and young adult patients.
“The cell and gene therapy fields are on the cusp of multiple regulatory approvals in the near term,” said Bruce Levine, Barbara and Edward Netter Professor in Cancer Gene Therapy in the Perelman School of Medicine at the University of Pennsylvania. “The challenges ahead lie in developing manufacturing and testing processes incorporating automation that can bring costs down and allow access to more patients.”
Developing broad-based innovations
Critical innovations often occur at the boundaries of disciplines, and CMaT will bring together relevant specialties for both research and workforce development, noted Madeline Torres-Lugo, a professor in the Department of Chemical Engineering at the University of Puerto Rico, Mayaguez Campus.
“Due to the complexity of cells as living organisms, a team with a strong background in biology, chemistry, physics, materials science, and engineering is required for this initiative,” Torres-Lugo said. “Our participation and contribution to CMaT will ensure that Puerto Rico not only remains at the forefront of pharma manufacturing, but also supports cell manufacturing technologies here and around the world by educating highly talented engineering students.”
CMaT testbeds have been selected to address several cell types that are in early stages of clinical adoption or moving toward clinical applications, but it isn't yet clear what cell types will have the greatest therapeutic impacts, noted Sean Palecek, the Milton J. and A. Maude Shoemaker Professor in chemical and biological engineering at the University of Wisconsin-Madison. Therefore, one of the center’s challenges will be to ensure that fundamental discoveries, and tool and technology development efforts, will apply to multiple cell types.
“Our work will provide safer and more potent cell products that will allow clinical studies to establish the effectiveness of these cells as therapeutics,” Palecek said. “In addition, our work on scaling cell production will enable manufacturing of sufficient numbers of cells to replace damaged organs, such as the loss of heart muscle after a heart attack, at a cost that makes these therapies accessible to broad segments of society. We will also train the future leaders of the emerging therapeutic cell manufacturing industry. These students and their work establishing this industry will be the most significant impact of CMaT.”
New centers among 19 ERCs
Since the program’s inception in 1985, NSF has funded a total of 74 ERCs and will support 19 in this fiscal year, including four new centers. Each center receives NSF funding for up to 10 years. During this time, centers build partnerships with industry, universities and other government agencies that will sustain them for years to come.
In May, the National Academies published a report, “A new vision for center-based engineering research,” which was the result of an NSF-funded study to examine the future of the NSF ERC program.
The report identifies and recommends strategies to enable NSF multidisciplinary engineering research centers to continue addressing key research, education and innovation needs of the United States in a changing global context.
“ERCs are widely known as outstanding examples of successful partnerships between universities, private industry and government that have made significant contributions to address national challenges,” said Don Millard, acting division director for the NSF Division of Engineering Education and Centers. “We are continually working with the scientific and engineering communities, as well as private industry and government partners, to ensure NSF-funded centers and grantees are best-equipped to match societal needs with research abilities.”
- John Toon, Georgia Tech News Center
Dr. Wayne Whiteman's online course Introduction to Engineering Mechanics made Class Central's list of Top 50 MOOCs of All Time for 2017. Introduction to Engineering Mechanics serves as an introduction to learning and applying the principles required to solve engineering mechanics problems. The course addresses the modeling and analysis of static equilibrium problems with an emphasis on real world engineering applications and problem solving.
Dr. Whiteman's course is one of only two engineering offerings in the Top 50. The list includes MOOCs (massive open online courses) from all disciplines, including computing, humanities and social sciences. Class Central, a giant curated catalog of MOOCs spread across different online course provides, created the list from over 8,000 MOOCs from around 750 universities worldwide, basing the rankings on thousands of reviews written by Class Central users.
The 2017 Top 50 list includes courses from 35 universities (22 of which are in the U.S.) and one research institute. Coursera is the top MOOC provider with 28 courses in the Top 50 and edX is second with nine courses.
By providing a well-reviewed list of offerings, Class Central hopes to encourage new learners to explore the world of online courses and facilitate a great first experience.
Dr. Shuman Xia was awarded the 2017 James W. Dally Young Investigator Award for his outstanding contributions to engineering education and excellence in research in experimental nano- and micro-mechanics.
The James W. Dally Young Investigator Award is given annually by the Society for Experimental Mechanics (SEM) to recognize a young professional (within 10 years of Ph.D.) who has made significant contributions in education and has demonstrated an excellence in research in the field of experimental mechanics.
Dr. Shuman Xia is an Associate Professor in the Woodruff School of Mechanical Engineering at the Georgia Institute of Technology. He obtained his B.S. in Theoretical and Applied Mechanics with highest distinction from Beijing University in 2003, and then attended Brown University where he received a M.S. in Applied Mathematics and a Ph.D. in Engineering (Solid Mechanics) in 2008. He joined the faculty of Georgia Tech in 2011 after completing postdoctoral studies at the California Institute of Technology.
His research interests include mechanics of energy storage materials, experimental nano- and micro-mechanics, fracture and failure of heterogeneous media, and mechanics of active materials. He is the recipient of the Orr Early Career Award from the Materials Division of the American Society of Mechanical Engineers (ASME), the Fylde Strain Best Paper Prize, and the NSF Faculty Early Career Development Award.
Woodruff School Associate Professor Dr. Ueda was recently awarded a $300K grant from the National Science Foundation to study dynamic robot vision inspired by the principles of human vision for effective image de-blurring and panoramic image stitching.
The project goal is to build a robotic vision system that mimics human eye motions. The research could lead to an enhanced vision system able to achieve quick scanning capability (saccadic eye motion) and smooth object following capability (smooth-pursuit) with minimal computational overhead while using inexpensive generic hardware. Current state-of-the-art systems require significant computer post-processing and expensive hardware.
Dr. Ueda's new NSF project will pave the way for a novel camera positioning system (hardware) and real-time vision (software) system that does not require any post-processing and produces ready-to-use images in real-time.
While previous studies focused solely on either mechanical design or image processing, Dr. Ueda’s research merges the areas of system dynamic and image processing. In coordination with inherently discrete and rapid ocular movements, the developed image processing methods inspired by ocular physiology mimic saccades and smooth-pursuit in a fast-moving robotic eye. The employment of a piezoelectrically driven robotic camera positioning mechanism will demonstrate the effectiveness of this ocular physiology-inspired approach.
Inventaprint, Inc., a new sponsor for the Woodruff School’s Capstone Design Course, has offered exclusive service to all Georgia Tech Capstone Design students. The company, started by Georgia Tech alumni Richard Mokuolu, BSME ’12, and Roland Mokuolu, BSCE ’12, connects inventors and businesses with vetted manufacturers to simplify the creative process and achieve lower manufacturing costs.
"As fellow Yellow Jackets, my twin brother, Roland Mokuolu, and I are excited to help democratize the innovative process by providing access to manufacturing,” says Richard. “That's why we founded Inventaprint. We look forward to help bring the students' innovative Capstone Design ideas to life!"
Inventors themselves, Richard and Roland knew first hand how hard it was to find a manufacturer who could accommodate lower volume orders, while still creating high -quality products. After continuing to run up against this problem, the brothers set out to track down the best manufacturers around the country—from the plains of Kentucky to Hong Kong. Through their search they found good, honest people who valued quality as much as they do and so Inventaprint was born.
Each Georgia Tech academic semester, Inventaprint will offer exclusive subscription discounts worth thousands of dollars, to full-time students in good standing participating in the Capstone Design course. Inventors upload their CAD files to job postings, initiating a bidding process where vetted manufacturers review files and offer recommendations on materials and tolerances based on their expertise. The process then allows inventors the opportunity to have their parts manufactured by a trusted builder that aligns with their vision and budget.
Manufacturers carry top-notch certifications and support both low and high volume orders. All manufacturer communication, drawing reviews, orders, re-orders, payment and shipping takes place on the Inventaprint platform and the intellectual property developed during the process remains the property of the inventor.
“We are excited regarding our partnership with Inventaprint,” said Dr. Amit Jariwala, Director of Design and Innovation at the Woodruff School. “We hope that their online platform will help our students gain an additional real-world experience in the process of transforming a design into a product.”
An interdisciplinary team from MIT and Georgia Tech has been awarded a $2.6M National Institutes of Health grant entitled "Scalable Cell- and Circuit- Targeted Electrophysiology."
Led by multiple PI’s Professor Edward Boyden (Associate Professor, Media Lab and McGovern Institute, Depts. of Biological Engineering and Brain and Cognitive Sciences, Massachusetts Institute of Technology) and Professor Craig Forest (Associate Professor, Woodruff School of Mechanical Engineering, Georgia Tech), the team will undertake a four-year effort (2017-2021) to empower scientists to characterize synaptic functions in vivo which could greatly accelerate our ability to understand how synapses change in disease, and to pinpoint new clinical targets. By enabling a robotic approach to characterizing synaptic properties, this proposal will empower high-throughput analyses of synapses in vivo.
The grant will enable individual investigators to rapidly analyze synapses between neurons exhibiting neural codes in a behavioral context, so that it is possible to understand how information is transformed at synapses. Researchers will invent a multiple-neuron image-guided whole cell patch clamp robot, to enable the simultaneous characterization of the neural codes in multiple neurons, as well as the synaptic connections between them. By miniaturizing the hardware, and incorporating a novel nanoscale anatomy exploration tool (expansion microscopy), investigators aim to create a powerful, easy-to-use toolbox that makes the critical task of in vivo synaptic physiology into a routine, automated procedure. By the end of this fast-paced 4-year technology development grant, the team will distribute all tools and datasets as freely as possible, sharing all algorithms, circuit designs, and assembly instructions, and hosting visitors to learn these technologies – for which they have an extensive track record.
The grant was awarded through the National Eye Institute (NINDS) of the National Institutes of Health (NIH) under Award Number R01NS102727.
Automation and Mechatronics, one of twelve Research Area Groups in the Woodruff School of Mechanical Engineering, has made the decision to change it's name to further support the group's vision, mission and strategy.
"The Automation and Mechatronics (A&M) Research Area Group is proud to announce the change of its RAG name to Automation, Robotics and Control (ARC). The RAG has a long history of conducting world-class research in system dynamics, machine design, and controls for advanced mechatronics and automation systems," says Dr. Jun Ueda, ARC's Research Area Group Chair. "Recently Robotics has become the RAG's focal area of research, particularly with the addition of the new faculty with robotics expertise. ARC faculty members are working closely with the Institute for Robotics and Intelligent Machines (IRIM). We hope that the new RAG name represents our faculty's contribution to this exciting field more precisely."
Research in the Automation, Robotics and Control area varies from fundamental research in control theory to the conception, design, and prototype evaluation of innovative mechatronics systems and applications to automation. Research in mechatronics focuses on the fusion of mechanical and electrical disciplines in modern engineering processes, aimed at achieving a cost-effective, optimal balance between mechanical structure and their overall control. Research topics include active and passive damping, adaptive learning and robust control of systems with uncertainty, automated manufacturing and demanufacturing, fuzzy and neural networks for control and identification, precision engineering and motion control, multimedia technology, intelligent sensors and acutators, vision-based motion control, and teleoperation.
Writer: John Toon
If you talk to Woodruff School alumnus David Anderson (MSME ‘12/PhD. ’15) about his time at Georgia Tech, he’ll tell you about the energy of being on campus and the unparalleled passion of the graduate experience. His stories are a testament to the hard work and focus of academic research and the lessons ingrained through his work at the George W. Woodruff School of Mechanical Engineering.
After receiving his bachelor’s in mechanical engineering from North Carolina State in 2005, Anderson went to work as a product development engineer for Caterpillar, the world’s largest construction equipment manufacturer. In 2010, the pursuit of a doctoral degree caught his interest and he found himself at Georgia Tech’s Woodruff School of Mechanical Engineering working with Dr. Andrei Fedorov for the duration of his master’s and PhD.
Anderson completed his thesis-based masters degree on an Air Force Research Lab project studying condensation on nanostructures with amphiphilic surfaces (i.e. surfaces that have a combination of hydrophilic and hydrophobic patterns). He then turned his sights to the problem of fuel cell vehicles and the prohibitive cost of developing a hydrogen delivery infrastructure. He wanted to find a way to produce hydrogen from natural gas using a catalytic reaction at a significantly lower temperature than commonly happens in the chemical industry right now. “What I was trying to do is say, hey, we have this natural gas distribution pipeline which makes it easier to transport natural gas than hydrogen,” he explained. “Could we essentially pipe the gas to the point of use, for example, the equivalent of a gas station for a fuel cell, and make the hydrogen fuel right there?”
A National Defense Science and Engineering Graduate Fellowship, awarded during his third year, resulted in funding for the project and enabled Anderson to pursue his PhD. Employing uniquely combined reaction and separation approaches in hopes of circumventing some of the thermo dynamic limits typically required to facilitate a reaction at high temperatures, he found a way to significantly reduce the reaction temperature to a point that would make his vision feasible.
Today Anderson’s focus is far broader. As senior engineer at Exponent, a multi-disciplinary engineering and scientific consulting firm, his work covers a variety of industries from medical devices and consumer products to oil and gas and even textiles. Being aware of what’s going on in several different fields allows him to see connections and bring an outside and unique perspective to diverse industry challenges, which can give rise to interesting solutions. “That's the nice thing about consulting at Exponent as compared to the big management consulting firms. I really am using fundamental science to solve unique technical problems, and getting to branch out into industries that I never thought I'd work in.”
He likes to describe Exponent as a kind of “rent-a-PhD” operation. Companies face numerous technical challenges that can arise at any time in any number of areas and it can be cost prohibitive to have someone on staff, full-time, with the level of capability and knowledge of a PhD. With a staff of over 700 consultants, more than half of which have PhDs in their fields, Exponent provides collaboration and expertise to solve challenging problems for short-term engagements.
Anderson was introduced to Exponent at a career fair on campus and found that the company was a natural fit for what he was looking for – that perfect blend of industry and academia. “I’m a very applied person, and I like solving urgent, immediate problems. I realized that at least in this stage of my life, the pure form of discovery in academia wasn’t what I wanted – but I also left industry because it was too routine and there wasn’t enough room for creativity. I like to refer to Exponent in terms of the children’s story Goldilocks– not too hot and not too cold. It’s right in the middle.”
Anderson credits the Woodruff School’s approach to curriculum, allowing students to pick their own academic paths, as the foundation for his ability to comfortably branch out into, and be open to, a variety of industries. “The nice thing about mechanical engineering is that it is so broad. I think as a mechanical engineer you are trained to be more of a generalist but then you can do deep dives into specific topics, and you have the capacity to become an expert. But you don't focus in on one solo path - everything stays out there for you. I definitely use the fundamental first principles and deep technical knowledge I learned at Tech, but beyond that I learned how to approach hard problems, think critically, and ask the right questions to generate insight and deal with uncertainty.”
Reflecting on his career thus far, Anderson acknowledges that he has become more adaptive and open to whatever path presents itself. Making a difference and an actual impact on people and the world in a general sense holds a great value. And for undergraduate and graduate students, he has some advice to impart. “If I could convey one concept to any student at Tech, this is it: You’re always a constantly evolving and developing person, so don’t underestimate your capacity to change and grow and develop. Do something you never thought you could see yourself doing. Whatever it is - maybe you think you're really at risk at first but under the right conditions you could develop into someone who could have a great idea and start a company. Just because you don't see that path right now doesn't mean that it's not there and that you aren't going to be able to find it eventually.”
At the end of the day, Anderson views mechanical engineering as a framework and a toolkit for solving the really interesting, pressing problems in the world. “It’s a way of looking at things and a way of approaching things to try to make them better – and it’s exciting! Ultimately, it’s a mindset of constant improvement.”